A vertical windmill, known technically as a Vertical Axis Wind Turbine (VAWT), is a wind-powered energy device in which the rotor shaft is oriented vertically.
Unlike traditional Horizontal Axis Wind Turbines (HAWTs), vertical turbines capture wind from all directions simultaneously, removing the necessity for orientation mechanisms like yaw controls.
Types of Vertical Windmills
Vertical windmills, also known as Vertical Axis Wind Turbines (VAWTs), primarily come in two distinct types: the Darrieus turbine and the Savonius turbine.
Both types differ fundamentally in design, operating principles, efficiency, and applications.
1. Darrieus Turbine
The Darrieus turbine, named after the French engineer Georges Darrieus, who patented the design in 1931, is characterized by its distinct, aerodynamic blade shape.
This turbine typically consists of two or three aerofoil-shaped blades vertically mounted on a central shaft, curving outwards in an eggbeater-like form.
Detailed Design Description
The blades of a Darrieus turbine have aerofoil cross-sections specifically engineered to maximize aerodynamic lift.
They are commonly constructed from lightweight and durable materials such as fiberglass, aluminum alloys, or carbon fiber composites.
The shape and angle of these blades significantly influence the turbine’s aerodynamic performance, directly impacting energy conversion efficiency.
Principle of Operation
The Darrieus turbine operates using aerodynamic lift, similar to an airplane wing.
When wind flows across the aerofoil-shaped blades, it creates a pressure difference between the blade’s two surfaces.
This pressure difference generates lift perpendicular to the direction of the wind flow, causing the blades—and thus the rotor—to spin.
Because lift forces become effective only when the turbine is already rotating, Darrieus turbines are generally not self-starting and typically require an external mechanism or motor to initiate rotation.
Efficiency and Performance
Darrieus turbines are known for relatively high efficiency among vertical axis wind turbines, typically achieving efficiencies ranging from 25% to 40%, particularly effective in moderate to high wind speed scenarios.
Due to their lift-based mechanism, these turbines can reach higher rotational speeds, making them well-suited for electricity generation at commercial scales.
Common Applications
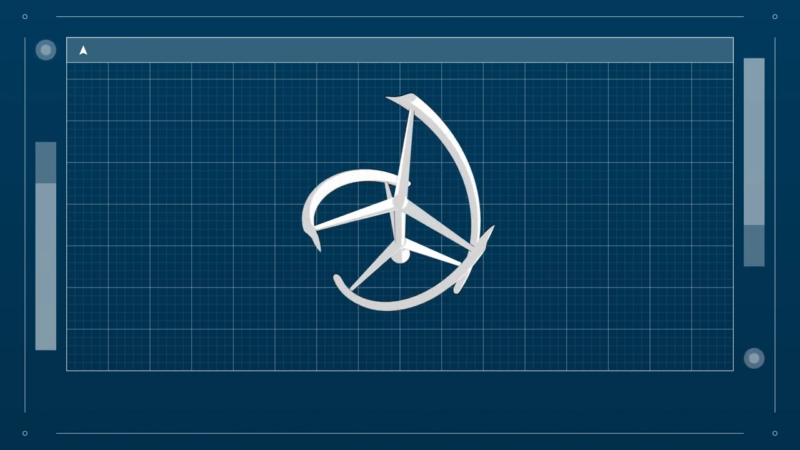
They are also viable in offshore wind farms because their vertical axis design can handle turbulent winds effectively without additional yaw control mechanisms.
Aspect
Darrieus Turbine Characteristics
Design features
Curved aerofoil-shaped blades vertically attached
Materials
Aluminum, fiberglass, carbon composites
Operating Principle
Aerodynamic lift from wind flow over aerofoil blades
Self-starting
Typically not self-starting
Efficiency
Typically 25–40%
Applications
Large-scale commercial, industrial, offshore wind farms
2. Savonius Turbine
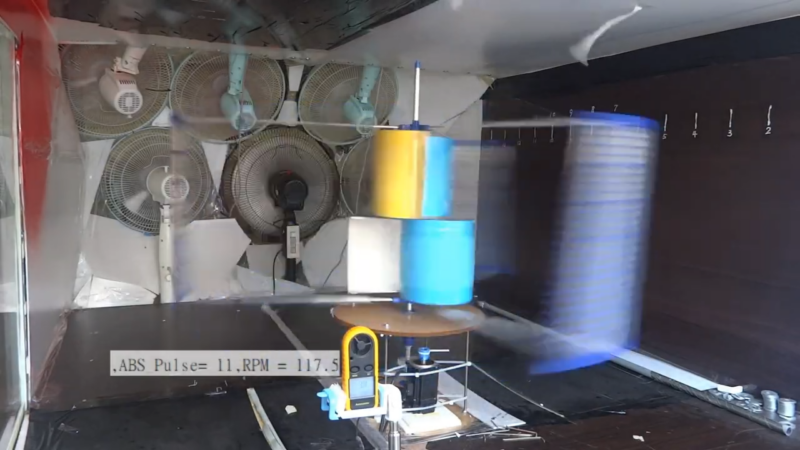
The Savonius turbine, invented by Finnish engineer Sigurd Johannes Savonius in the early 1920s, is notably different in form and operational principle compared to the Darrieus turbine.
Its design emphasizes simplicity, ease of manufacturing, and reliability, particularly suitable for conditions with variable and lower wind speeds.
Detailed Design Description
The Savonius turbine consists of vertically oriented, semi-cylindrical blades shaped like scoops, typically arranged in pairs facing opposite directions, forming an “S” shape when viewed from above.
The blades can be made from various materials, including steel, aluminum, plastic, or recycled materials.
Due to their straightforward geometry, Savonius blades are easy to construct, install, and maintain.
Principle of Operation
Unlike the Darrieus turbine, the Savonius turbine operates purely through aerodynamic drag forces.
When wind hits the concave side of the blades, it exerts a greater force on the blade than on the convex side, creating torque that turns the rotor.
This drag-driven mechanism enables the Savonius turbine to begin rotating even at very low wind speeds without any external starting system.
Efficiency and Performance
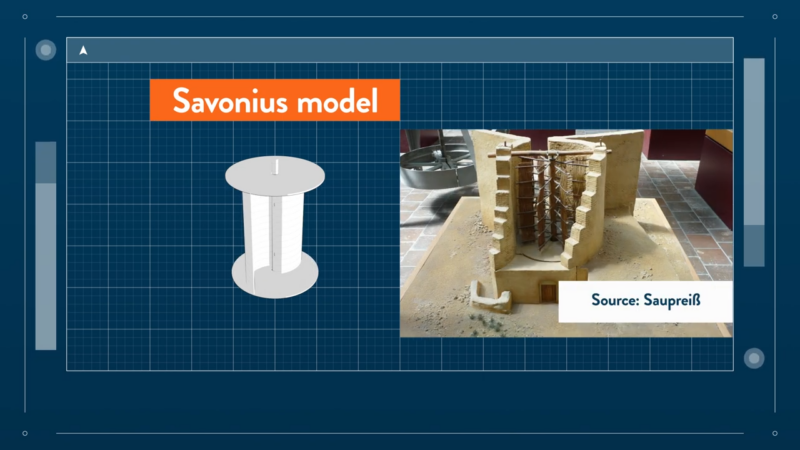
Although less efficient, they operate effectively under low wind speed conditions (as low as 2 to 3 meters per second) and offer excellent reliability and consistent energy generation in regions with turbulent or inconsistent winds.
Common Applications
The straightforward design, robustness, self-starting capability, and reliability at low wind speeds make the Savonius turbine ideal for residential, small-scale commercial, remote off-grid applications, rural electrification projects, and portable wind generation systems.
Aspect
Savonius Turbine Characteristics
Design features
Vertically oriented, semi-cylindrical scooped blades
Materials
Steel, aluminum, plastics, recycled composites
Operating Principle
Aerodynamic drag; wind pushes blades creating torque
Self-starting
Self-starting
Efficiency
Typically 15–20%
Applications
Residential, small-scale commercial, remote locations, low-wind environments
Detailed Operation and Mechanisms
Vertical windmills convert kinetic energy from wind into electrical energy through a clearly defined and systematic process, involving several distinct phases:
1. Interaction of Wind with Turbine Blades
The process begins when wind interacts with the turbine blades, causing rotation. Depending on the type of vertical windmill, this interaction can be fundamentally different:
- In the Darrieus turbine, wind flowing over the curved, aerofoil-shaped blades creates aerodynamic lift forces, causing rotation. These lift forces arise due to a difference in air pressure generated as air moves faster over one side of the blade than the other.
- In the Savonius turbine, wind directly pushes against the semi-cylindrical blades, generating drag forces. The wind exerts greater pressure on the blade surfaces facing the wind, resulting in torque that initiates rotation even at low wind speeds.
2. Rotation and Rotor Movement
The rotor, consisting of blades attached to a vertical shaft, begins to spin due to wind interaction.
The rotation speed of the rotor depends on blade design, turbine type, wind velocity, and rotor size.
As rotation occurs, kinetic wind energy transforms into mechanical rotational energy, transmitted directly or indirectly (via gears or a gearbox in some installations) to the main vertical shaft.
3. Conversion of Mechanical Energy to Electrical Energy
The mechanical energy from the rotating vertical shaft is transferred to a generator, typically attached at the base or midpoint of the turbine structure.
This generator converts mechanical rotational energy into electrical energy through electromagnetic induction.
When the shaft rotates within the generator, it induces electrical current in coils of wire surrounding magnetic fields. The produced current initially is often direct current (DC).
4. Electrical Regulation and Distribution
The generated electrical energy typically requires regulation before it becomes usable.
Initially, it may pass through a charge controller or voltage regulator to stabilize output voltage and current.
Then, this regulated electricity goes through an inverter system, which converts DC electricity into alternating current (AC). The AC power generated can be:
- Immediately supplied to local homes, businesses, or other consumers.
- Distributed to the electrical grid.
- Stored in batteries or energy storage systems for later use, particularly in remote or off-grid installations.
Comparative Analysis: Vertical Axis (VAWT) vs. Horizontal Axis (HAWT)
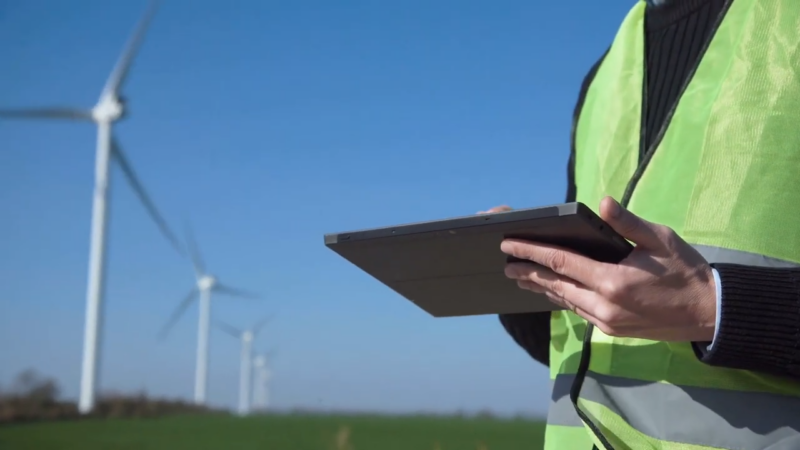
A detailed comparative analysis is essential for understanding their suitability under various conditions and applications, according to Research Gate:
Parameter
Vertical Axis Wind Turbine (VAWT)
Horizontal Axis Wind Turbine (HAWT)
Efficiency (typical)
25–40% (Darrieus), 15–20% (Savonius)
40–50%
Directional Dependence
Omnidirectional (no yaw mechanism needed)
Direction-dependent (requires yaw mechanism)
Maintenance Complexity
Lower (simpler mechanisms, fewer moving parts)
Higher (complex yaw mechanisms, gearbox maintenance required)
Noise Levels (at 100m)
30–40 dB (quiet operation suitable for residential/urban areas)
40–50 dB (higher noise, less urban-friendly)
Wind Speed Performance
Effective in low-to-moderate winds (Savonius: 2–10 m/s, Darrieus: 6–15 m/s)
Optimal in stable, moderate-to-high winds (8–15 m/s)
Urban Suitability
High (compact, quiet, visually adaptable)
Lower (larger structures, more noise, visual impact)
Efficiency and Performance
Vertical windmills generally exhibit lower efficiency compared to horizontal turbines. Typical efficiency values for VAWTs are approximately 25-40% for Darrieus-type turbines and 15-20% for Savonius-type turbines.
In contrast, HAWTs achieve higher efficiencies, typically ranging from 40-50%. This efficiency gap arises primarily because horizontal axis designs are optimized specifically for steady, consistent winds.
Directional Dependence and Operational Complexity
One key advantage of vertical turbines is their omnidirectional nature. Vertical windmills do not require complex orientation mechanisms (yaw control systems), as they efficiently capture wind from any direction.
In contrast, horizontal turbines must actively adjust their orientation to face directly into the wind, requiring sophisticated yaw mechanisms and gearboxes, thereby increasing complexity and maintenance requirements.
Maintenance Complexity and Cost Implications
VAWTs generally require lower maintenance than HAWTs, mainly due to their simpler structural designs and absence of yaw mechanisms.
Reduced mechanical complexity typically results in lower operational costs and fewer points of mechanical failure, making vertical turbines particularly advantageous in remote or offshore locations.
Noise Levels and Environmental Impact
Vertical turbines typically produce lower noise levels (approximately 30-40 decibels at 100 meters) compared to horizontal turbines (40-50 decibels at 100 meters).
Lower noise emissions significantly enhance their suitability for installation in urban, suburban, or noise-sensitive locations.
Wind Speed Suitability
Vertical turbines, particularly Savonius types, are effective in low wind conditions (wind speeds as low as 2–3 m/s), whereas Darrieus types perform optimally at moderate-to-high wind speeds (approximately 6–15 m/s).
Horizontal turbines generally require higher, stable wind speeds to operate optimally, typically from 8–15 m/s, which limits their practical use in areas with fluctuating or low wind speeds.
Suitability for Urban and Residential Use
Vertical turbines have a higher suitability for urban environments due to their compact size, reduced noise, and aesthetic adaptability. Horizontal turbines, while highly efficient, typically have a larger visual footprint, produce higher noise, and thus are less practical in densely populated urban areas.
Relevant Statistics and Market Data
Vertical Axis Wind Turbines (VAWTs) vary significantly in operational efficiency based on their specific type and design features.
The efficiency of these turbines directly influences their suitability for different wind conditions:
Average Efficiency
The efficiency of vertical wind turbines varies notably between the two primary turbine types:
Turbine Type
Average Efficiency (%)
Optimal Wind Speed Conditions (m/s)
Common Applications
Darrieus
25–40%
Moderate to high wind (6–15 m/s)
Commercial, industrial, offshore installations
Savonius
15–20%
Low wind speeds (~2–10 m/s)
Residential, remote, small-scale installations
Global Installation Statistics (2024)
According to the latest renewable energy data (BloombergNEF, 2024), vertical axis wind turbines represent approximately 15% of the global small-scale wind energy market. This indicates steady but niche adoption within the broader wind energy industry, particularly in urban, residential, and remote applications where vertical windmills offer distinct advantages, such as reduced noise and easier maintenance.
The vertical windmill market is experiencing consistent growth, driven by increasing urbanization and global demand for decentralized renewable energy solutions.
The global vertical windmill market is projected to expand significantly over the coming years, with an expected compound annual growth rate (CAGR) of approximately 11.5% between 2023 and 2030.
Global Market Forecast (2023-2030)
The following table provides a detailed market forecast based on BloombergNEF’s renewable energy market outlook:
Year
Global Market Value (USD Millions)
Annual Growth Rate (%)
2023
2,900
–
2025
3,500
10.3%
2027
4,600
11.5%
2030
7,300
13.7%
Scientific Studies and Research Highlights
Several significant scientific studies have analyzed vertical windmills, confirming their unique benefits and practical viability in specific contexts:
Sandia National Laboratories Study (2019)
Sandia’s research confirmed the notable advantages of vertical turbines in offshore wind energy applications. Key findings include simplified maintenance due to fewer moving parts, the absence of yaw control systems, and enhanced stability in turbulent wind environments, making vertical axis turbines potentially more cost-effective and reliable for offshore installations compared to horizontal turbines.
MIT Urban Wind Study (2022)
MIT’s comprehensive urban wind study found vertical turbines to be highly suitable for city-based installations. According to the study, vertical windmills offered critical benefits such as reduced operational noise, minimal visual obstruction, and the capacity to function effectively in highly variable and turbulent urban wind conditions. MIT researchers suggest integrating these turbines on urban rooftops or small commercial buildings for local power generation.
Delft University of Technology Study (2020)
A detailed research study from TU Delft highlighted superior performance characteristics of vertical wind turbines in urbanized or densely populated areas. Specifically, the research emphasized the adaptability of vertical turbines to variable wind patterns common in urban environments. The study confirmed that vertical windmills notably reduce noise pollution and are well-suited for integration into urban infrastructure, contributing significantly to sustainable urban energy initiatives.
Practical Applications and Usage
Vertical windmills have been effectively integrated into various contexts globally due to their unique operating characteristics, reliability, and suitability for specific environments:
Application Area
Detailed Explanation
Residential Installations
Ideal for generating electricity at individual homes or small communities due to their compact size, quiet operation, and effectiveness at low wind speeds.
Urban Rooftops
Highly suitable for rooftop installation in cities, offering minimal visual disruption, noise control, and stable operation in variable urban wind conditions.
Offshore Wind Farms
Offer advantages in simplified maintenance, increased structural stability, and resilience in turbulent wind conditions, potentially lowering long-term operational costs.
Remote and Rural Areas
Provide reliable and consistent electricity generation in remote or off-grid locations, requiring minimal maintenance and operating effectively even at very low wind speeds.
Challenges and Limitations
Despite their benefits, vertical windmills face several challenges and limitations that currently restrict broader adoption, particularly at larger commercial scales:
Limitation
Explanation of Challenges
Efficiency Gap
Vertical windmills, especially Savonius-type turbines, have lower aerodynamic efficiencies compared to horizontal turbines, limiting their suitability for large-scale power generation.
Scaling Issues
Structural stability and aerodynamic constraints complicate scaling vertical turbines to larger sizes. Large-scale installations pose significant engineering challenges, reducing cost-effectiveness at commercial utility scales.
Initial Investment Costs
High upfront costs associated with advanced materials (e.g., carbon composites) and precision engineering required for efficient aerofoil blades, making vertical windmills more expensive initially compared to traditional solutions.
Conclusion
Vertical windmills represent a valuable segment within renewable energy technologies, characterized by their lower noise production, simpler maintenance requirements, and adaptability to diverse wind conditions, particularly in urban and residential contexts.
Current market trends and research clearly indicate growing adoption, especially in decentralized, urban, and remote applications, as technological advancements continue to improve efficiency and reduce manufacturing costs.
Nevertheless, critical challenges remain, particularly regarding efficiency improvements and scalability.
Addressing these limitations through continued research, development, and innovation will determine the broader applicability of vertical windmills in global renewable energy strategies.
Given ongoing developments and positive market forecasts, vertical windmills are poised for an increasingly significant role in sustainable and distributed energy generation solutions over the next decade and beyond.